Fluid Movement
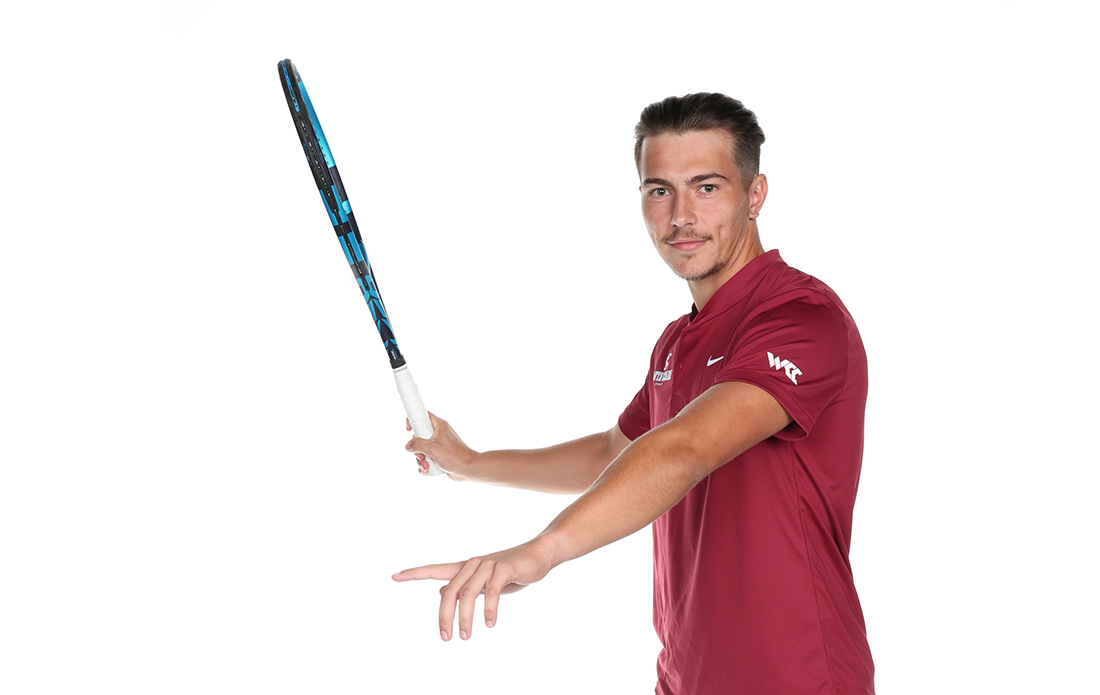
The movement involved in swinging a tennis racket is one of the most complex in biomechanics. A standard overhead serve has eight different stages and engages 15 muscles at different rates, each representing a potential data point.
When this algorithm of athleticism comes together just right, it’s art. But if it’s off, even by a little bit, muscles and tendons slowly work against each other, which can result in lowered performance and even injury.
Throughout his amateur and college tennis career, Nick Cmager ’23, M.S. ’24 spent hundreds of hours trying to crack this code. Starting in high school, he studied the reaction time of athletes from different sports to see if visual or sound stimuli could help him refine workouts.
“I was analytical about how I trained,” Cmager says. “I started tracking different parameters of my training and even developed my own technologies. I once made my own ice bath out of a deep freezer to have better recovery.”
Now, as a graduate bioengineering student and former collegiate tennis player at Santa Clara, he’s using what he’s learned in class to help everyone from professional athletes to weekend warriors get the most out of their bodies.
Thanks to funding from the National Science Foundation (NSF), Cmager is working with associate professor of bioengineering Emre Araci to develop a system of microfluidic and skin strain sensors that track how frequently, and to what degree, a muscle is engaged during a workout or over a day.
“A lot of tracking technologies on the market come with drawbacks,” Cmager explains. “Motion capture needs to be done in a controlled environment in a lab with cameras arranged neatly. Epidermal electronics can be non-stretchable, which is uncomfortable on the skin. Microfluidics is an innovative option.”
Cmager’s microfluidic devices, shaped a bit like a temporary tattoo or nicotine patch, feature extremely small micro-channels of liquid that run like a circuit along the surface of the patch. As muscles flex and skin is stretched or “deformed,” small amounts of fluid advance through the micro-channels. The more the muscle is engaged, the more the fluid progresses. Once the patch is removed, the movement can be tracked by a computer.
When multiple patches are arranged strategically on the body, the results are triangulated and data can be tied to certain movement sequences. This data could be used in a variety of ways, Cmager says, from refining an athlete’s technique on the field of play to improving form in training and rehabilitation.
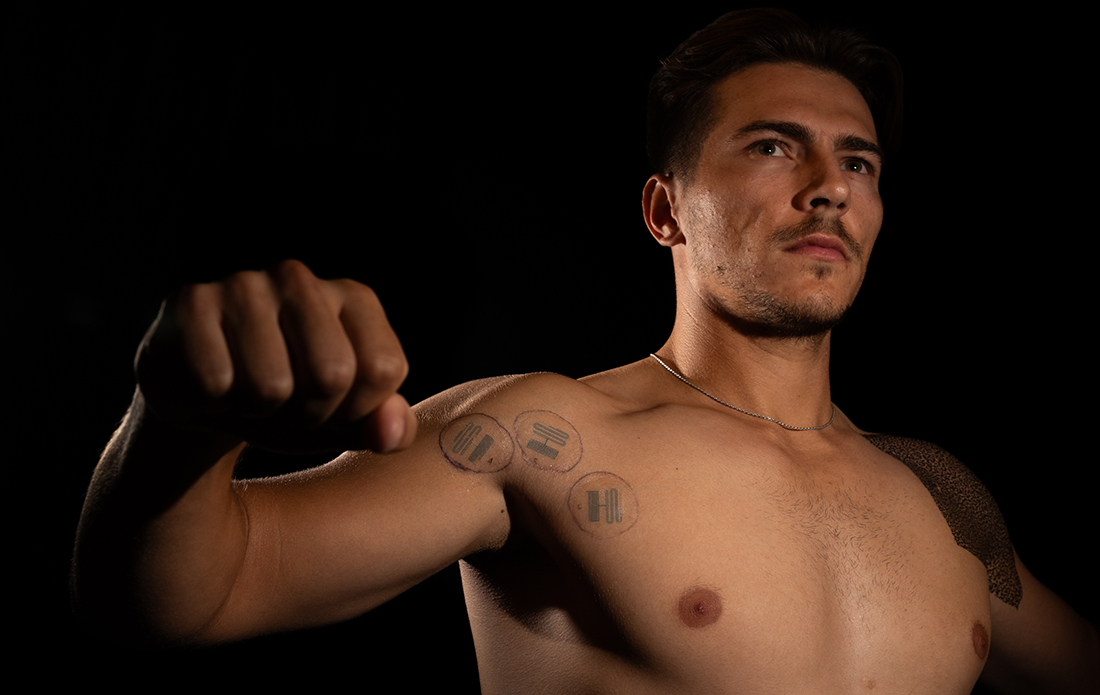
When positioned strategically, microfluidic sensors can track specific movements | Photo by Jim Gensheimer
Cmager points out that it's not uncommon for a collegiate team of 10 or more athletes to perform different exercises across a weight room simultaneously. With only one strength and conditioning coach, there can be an elevated risk of improper form or injury.
“Squats for example are very technical, and if you're not doing them right, you can easily get injured,” Cmager explains. “Having one coach with 10 players, it's hard to monitor them all. So if you have sensors that can make sure everyone's performing the movements correctly, that has a huge value.”
Cmager also envisions these devices to be used with physical therapy at home. Physicians could prescribe rehab exercises and use the devices to help assess the quality of the movements when they're not around. Or, doctors could monitor muscle engagement over the course of a day to identify overuse that could be causing an injury.
Perhaps most impressive, the data could eventually be used to refine the skills of aspiring athletes, Cmager says. For example, if a tennis player is struggling with velocity or accuracy on their serve, the sensors could detect that they’re swinging too far over their shoulder or with an overly bent elbow, even if just by a fraction.
“We tested our sensors on the shoulder and were able to differentiate between three different unweighted movements: shrug, abduction, row,” Cmager explains. “For more complex movements like the serve, we’ll need to tailor our devices for more specific applications and make sure our manufacturing is consistent. But detecting more complex moves is definitely our goal.”
Araci first explored microfluidic technology five years ago when he developed a contact lens to monitor eye pressure for glaucoma patients. Patients would wear the clear contact lens and if their eye pressure changed, the fluid would move and could be monitored using an app on their phone.
Araci says this is a continuation of that research, but the science is even more complex.
“That was a simple device. You had a circularly symmetric device placed on a sphere,” Araci explains. “Now, we have very complex patterns that are created on the skin. You need to know what those patterns look like when performing the exercise. Then you need to have a network of sensors placed accurately on the skin so you can measure one type of movement.”
This is Cmager’s second research project designed to improve athletic rehabilitation. As part of his Senior Design project last year, he collaborated with two Santa Clara University soccer players to use EMG sensors to create a more affordable and reliable way to perform strength testing for injured athletes.
Cmager is finishing up his master’s degree this spring and applying for jobs in the medical device industry but is still hard at work on his research. He and Araci recently filed a patent for the microfluidic technology and are finishing up a research paper on their findings, which they plan to publish in a scientific journal.
While they’re still relatively early in their research, they believe the applications of this technology could be wide-ranging, even outside of athletics, including skin-drug delivery applications for a variety of conditions.
“In addition to tracking movement, the sensors could even store and administer a drug,” Cmager says. “When the person moves, the more movement the person does, the more of the drug gets delivered to the person.”
Nanoscale science and technology is a new frontier in engineering that deals with the creation of functional materials, devices, and systems at a scale of less than one hundred nanometers to one ten-thousandth of a millimeter. The Center for Nanostructures (CNS) pursues interdisciplinary research and education through partnerships with other universities, industry, and government.
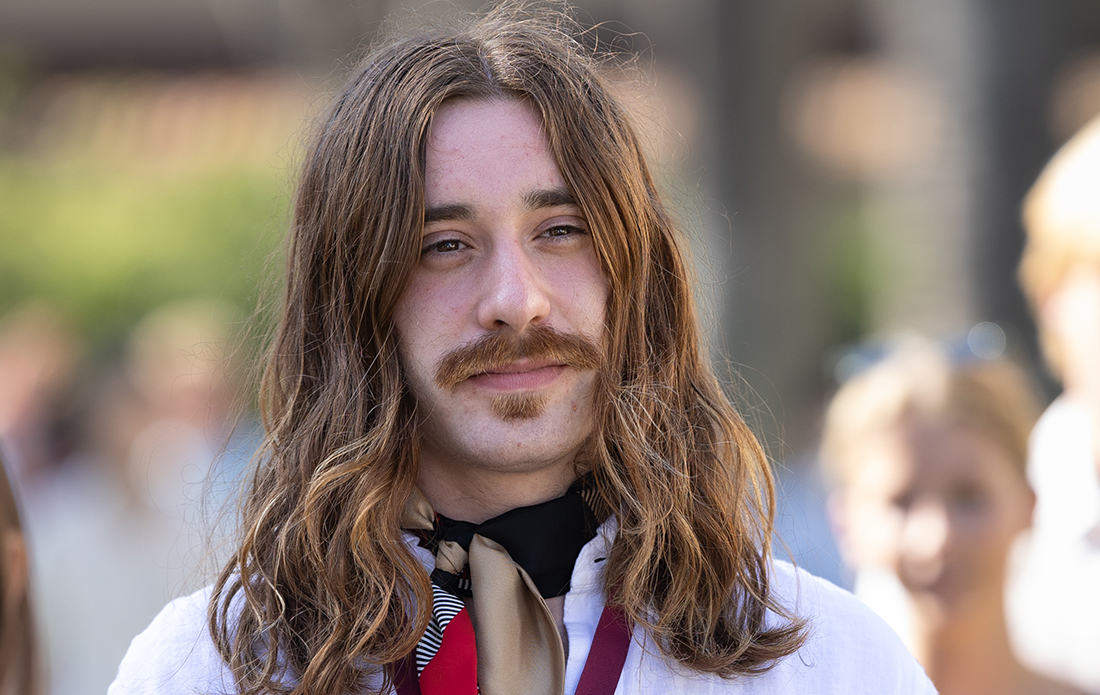
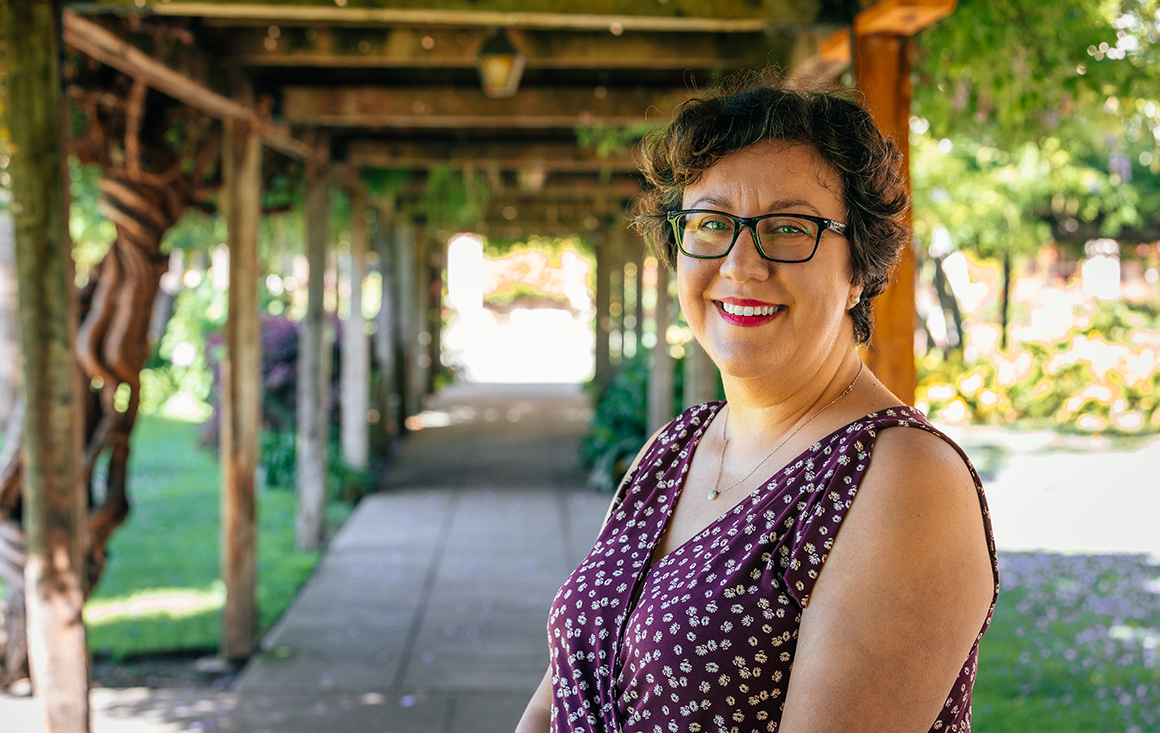
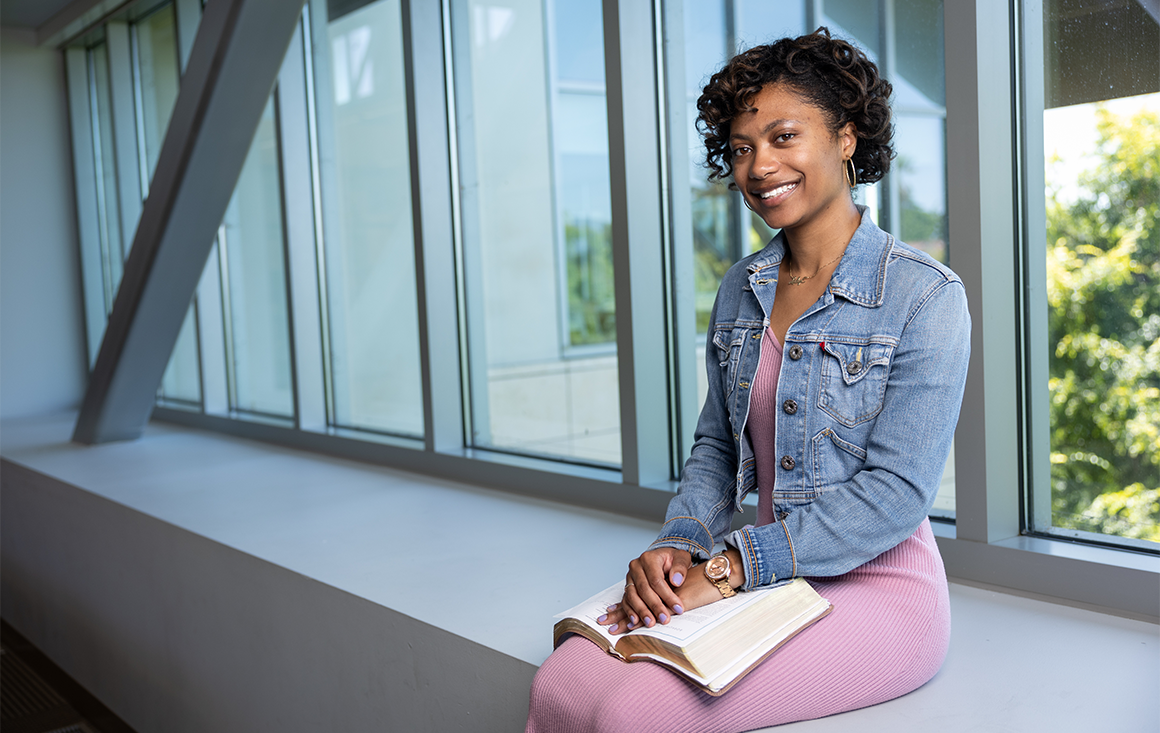